Have you ever heard about supersymmetry?
- Alexa Ines Guido
- Apr 1
- 4 min read
Hola! I'm Alexa Guido, a young and curious woman passionate about science. Join me on an exciting journey to explore the wonders of the universe through the lens of physics.
Imagine for a moment that we suddenly doubled the number of fundamental particles in the Standard Model. Sounds mind-boggling, right? Yet, becoming the realm of particle physics two times more complicated is an idea not that far from our reality. While the Standard Model has proven to be remarkably successful in explaining the universe, physicists agree that it's incomplete, and that’s where supersymmetry steps in!
In simple terms, this theory proposes that for every known fundamental particle, there exists a partner particle. While this might seem extravagant, these partner particles could address various shortcomings in current scientific knowledge, such as the source of the mysterious dark matter in the Universe, the “unnaturally” small mass of the Higgs boson, the anomalous way that the muon spins and and even shed light on the unification of the fundamental forces of nature.

Now, let’s talk about symmetry. Symmetry is something we encounter daily, from glancing at our reflection in the mirror to examining the uniformity of a sphere. Small alterations can disrupt symmetry; for example, a face with a mole on one cheek breaks left-right symmetry. In physics, symmetry refers to the property of particles that remain unchanged under certain transformations. Moreover, every physical law remains valid in the universe at all places and times.
When physicists discuss symmetry, they often refer to how nature behaves when particles are swapped with their anti-particles (called “charge conjugation”), when coordinates are reversed as in a mirror (called “parity inversion”), or when time is reversed (running the “movie” backwards). These three types of symmetries are called C (for charge), P (for parity), and T (for time). Together, they form the concept of CPT symmetry, which operates in discrete steps, it happens in steps.
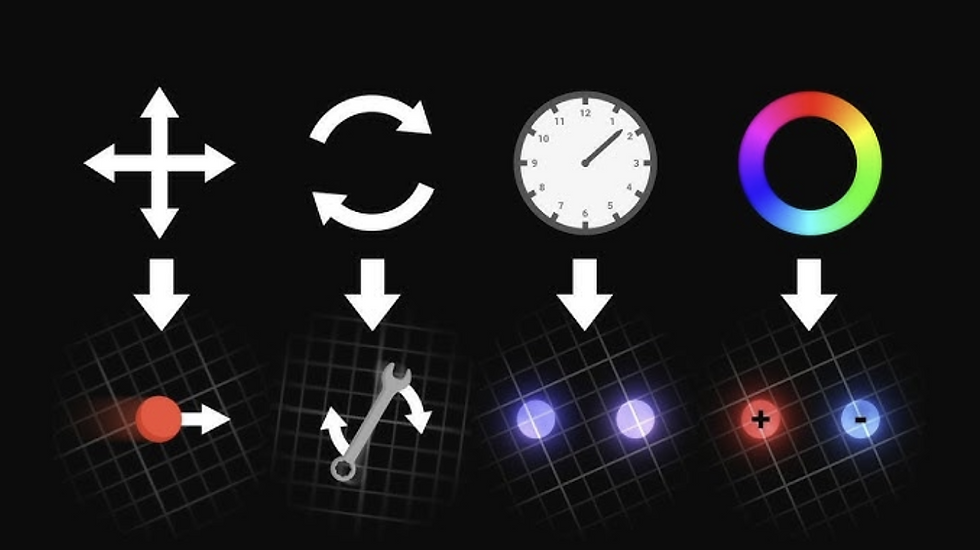
Physicists contrast discrete symmetry with continuous symmetry, where there are no steps between changes in symmetry. Take rotational symmetry, for example, when you rotate a circle, it remains symmetrical at every angle, unlike a square, which has specific points where its symmetry breaks.
For scientists, translational symmetry in time and space are especially important. This principle means that the laws of nature measured by one observer at one moment and place will hold true for another observer at a different time and location. Without this symmetry, the physical sciences and the universal laws of nature wouldn’t work, experiments wouldn't be reproducible, and science would be impossible.
So, what does this have to do with supersymmetry? As we mentioned earlier, the Standard Model seems to predict that all particles should be massless, an idea that contradicts our observations. Theorists have come up with a mechanism to give particles masses that requires the existence of a new particle, the Higgs boson. However, it is a puzzle why the Higgs boson should be light, as interactions between it and Standard-Model particles would tend to make it very heavy.
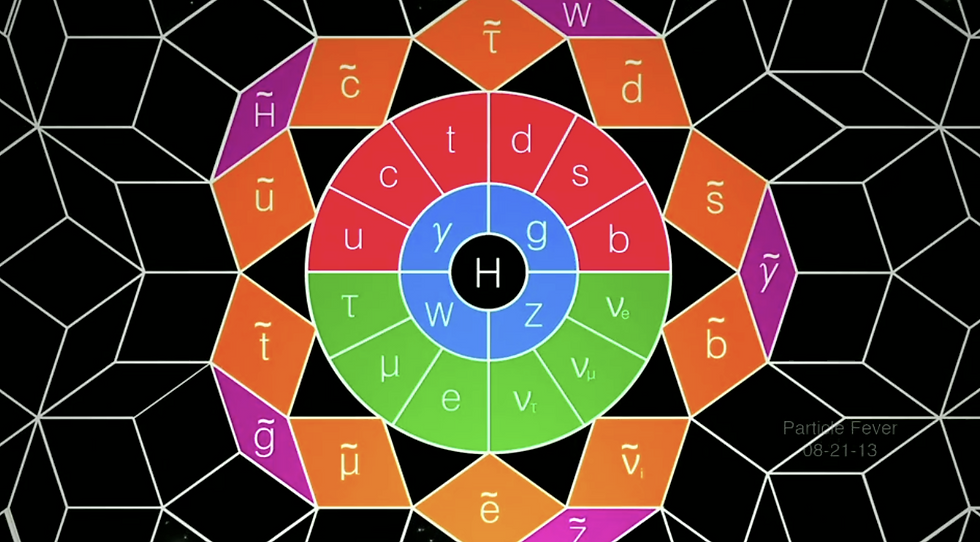
Here’s where supersymmetry comes into play. The additional particles it predicts could effectively counterbalance the contributions to the Higgs mass from their Standard Model counterparts, making a light Higgs boson possible. The new particles would interact through the same forces as Standard-Model particles, but with distinct masses.
If supersymmetric particles were included in the Standard Model, the interactions of its three forces – electromagnetism and the strong and weak nuclear forces – could have the exact same strength at very high energies, as in the early universe. A mathematical framework that achieves this unification of forces is termed a grand unified theory, and it’s the holy grail of physicists everywhere!
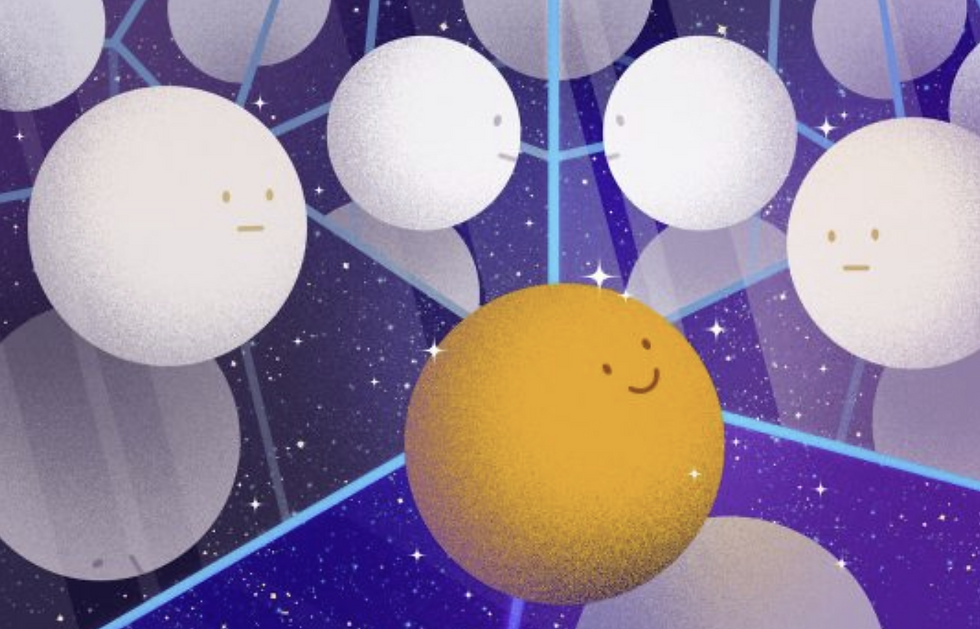
In essence, supersymmetry states that each particle in the Standard Model has a superpartner whose spin differs by half a unit. Fermions possess half a unit of spin, while bosons range from 0 to 2 units of spin. Thus, bosons are linked with fermions and vice versa.
Linked to their differences in spin are differences in their collective properties. Fermions are very standoffish; everyone must be in a different state, whereas bosons are more sociable, preferring to occupy the same state. Though fermions and bosons appear vastly different, supersymmetry brings these two categories together in a beautiful, unified framework.
These superpartners are represented here with a tilde (~). Fermions get bosons as superpartners, and bosons get associated with fermions. This unifies the building blocks of matter with the force carriers. Everything becomes more harmonious and symmetric.
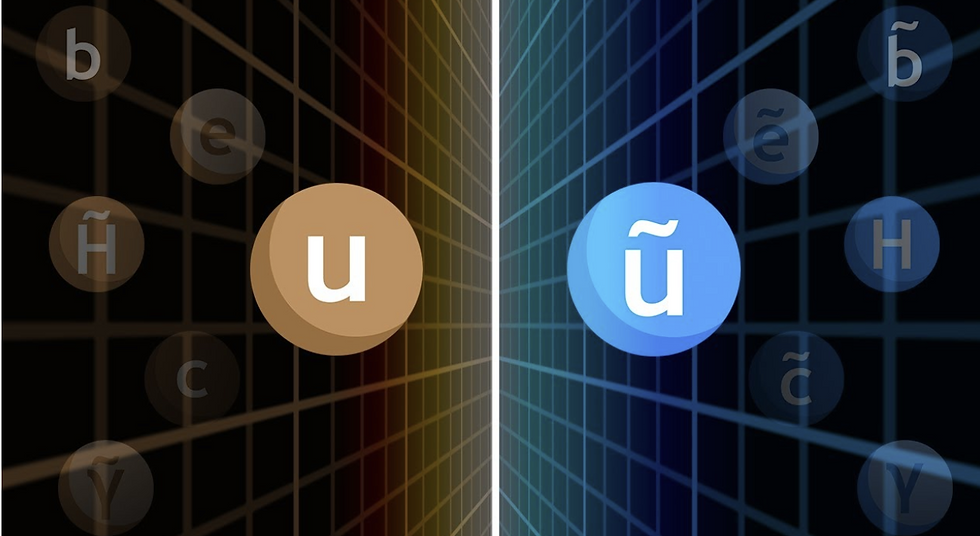
But there are other important consequences. Starting from the fact that the number of existing fundamental particles doubles, many of these partners can mix, giving combined states such as charginos and neutralinos
This has significant implications. For instance, the two superpartners to the top quark, called the stops, can cancel out the large contribution from the top quark to the mass of the Higgs boson. Moreover, the lightest supersymmetric particle, typically a neutralino (one of the mixed states with no electric charge), possesses the very characteristics we associate with dark matter.

Not only would supersymmetry fix the flaws of the Standard Model, it would also solve the dark matter problem. Killing two huge birds with one simple stone. But here’s the catch: if these supersymmetric particles truly exist, why haven’t we found any yet? The search continues, keeping the excitement of particle physics alive and undefined.
Sources:
Images:
Comments